James Clerk Maxwell, a Scottish physicist, made an indelible mark on the field of physics with his profound contributions, most notably his theory of electromagnetism. Born in 1831, Maxwell’s work bridged the gap between the mechanical and the electromagnetic, a leap that would fundamentally alter our understanding of the physical world. In 1865, through “A Dynamical Theory of the Electromagnetic Field,” Maxwell introduced a set of equations that not only unified the then-understood phenomena of electricity and magnetism but also predicted the existence of electromagnetic waves—ripples in the electromagnetic field that move through space at the speed of light. This theoretical prediction of radio waves, entities unseen and unexplored at the time, underscored the broader significance of electromagnetism beyond the confines of visible light and paved the way for future explorations into the electromagnetic spectrum.

https://commons.wikimedia.org/wiki/File:James_Clerk_Maxwell_statue_in_George_Street,_Edinburgh_03.jpg
Maxwell’s equations described how electric and magnetic fields are generated by charges, currents, and changes in each other. The most revolutionary aspect of his theory was the prediction that a time-varying electromagnetic field could propagate through empty space as waves. This was a startling revelation because it suggested that electromagnetic interactions could occur over vast distances without the need for a physical medium, challenging the prevailing wave theories of light that depended on the luminiferous ether. Maxwell’s work thus provided a theoretical framework that not only unified electrical and magnetic phenomena but also hinted at the vast, untapped potential of the electromagnetic spectrum for communication and other applications. The eventual experimental validation of Maxwell’s predictions about electromagnetic waves marked the dawn of the modern era of physics and telecommunications, highlighting his status as a pivotal figure in science.
- Background: The Path to Discovery
- Early Work in Electromagnetism
- Maxwell’s Inspiration
- Maxwell’s Theory of Electromagnetism
- The Formulation of Maxwell’s Equations
- Predicting the Existence of Radio Waves
- The Conceptual Leap
- Experimental Validation
- Heinrich Hertz’s Contribution
- The Impact of Hertz’s Experiments
- Interdisciplinary Echoes: Maxwell’s Equations Across Physics
- Similarities in Other Fields
- Fluid Dynamics
- Quantum Mechanics
- The Universality of Maxwell’s Work
- Inventions and Discoveries Stemming from Maxwell’s Work
- Radio Technology
- Radar and Microwave Technology
- Quantum Electrodynamics (QED)
- Conclusion
- Further Exploration
- Further Reading
Background: The Path to Discovery
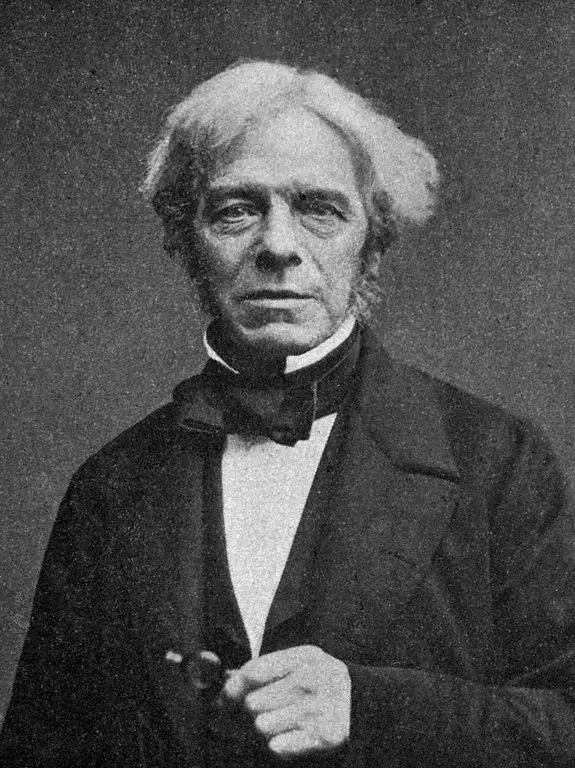
The journey towards James Clerk Maxwell‘s groundbreaking prediction of radio waves was paved by the foundational work in electromagnetism by several eminent scientists, among whom Michael Faraday stands out prominently. Faraday’s experimental work in the early 19th century significantly deepened the understanding of electromagnetic fields, setting the stage for Maxwell’s theoretical insights.
Early Work in Electromagnetism
Michael Faraday, an English scientist, made seminal contributions to the understanding of electromagnetism. Faraday’s law of electromagnetic induction, discovered in 1831, demonstrated that a changing magnetic field could induce an electric current in a conductor. This discovery was crucial for the later development of electric generators and transformers. Faraday also introduced the concept of the electromagnetic field, proposing that magnetic forces could be transmitted through space via these fields, a revolutionary idea that challenged the prevailing notion of action at a distance.
Faraday’s experiments with magnetic lines of force provided a tangible way to visualize electromagnetic fields, illustrating how they interacted with materials and currents. His work on electrolysis further established the quantitative relationship between electricity and chemical change, laying the groundwork for electrochemistry.
Maxwell’s Inspiration

James Clerk Maxwell was profoundly influenced by Faraday’s experimental discoveries and his intuitive grasp of field theory. Maxwell was particularly captivated by Faraday’s lines of force and sought to describe these phenomena mathematically. Where Faraday provided a physical and visual understanding of fields, Maxwell aimed to establish a rigorous mathematical framework that could describe the dynamics of these fields in space and time.
Maxwell’s approach was to use Faraday’s field concept as the foundation for his own theory, translating Faraday’s intuitive descriptions into precise mathematical language. Maxwell’s equations, formulated through this endeavor, encapsulated the principles of electromagnetism in a set of differential equations. These equations described how electric and magnetic fields are generated and altered by each other and by charges and currents. They also implied that electromagnetic fields could propagate through empty space as waves, a direct extension of Faraday’s work on fields.
In essence, Maxwell’s theoretical achievements were a monumental synthesis of the insights provided by Faraday and other researchers. By incorporating the concept of the electromagnetic field into a coherent mathematical framework, Maxwell not only unified the known phenomena of electricity and magnetism but also predicted new phenomena, such as electromagnetic waves, that would transform our understanding of the physical world. This blend of experimental groundwork and theoretical brilliance exemplifies the collaborative nature of scientific discovery, illustrating how theoretical advancements often stem from the fertile ground prepared by experimentalists.
Maxwell’s Theory of Electromagnetism
James Clerk Maxwell’s formulation of the electromagnetic field theory was a monumental achievement in physics, marking the dawn of a new era in understanding light, electricity, and magnetism. His theory is encapsulated in four fundamental equations, now known as Maxwell’s equations, which describe how electric and magnetic fields are generated and interact with each other and with matter.
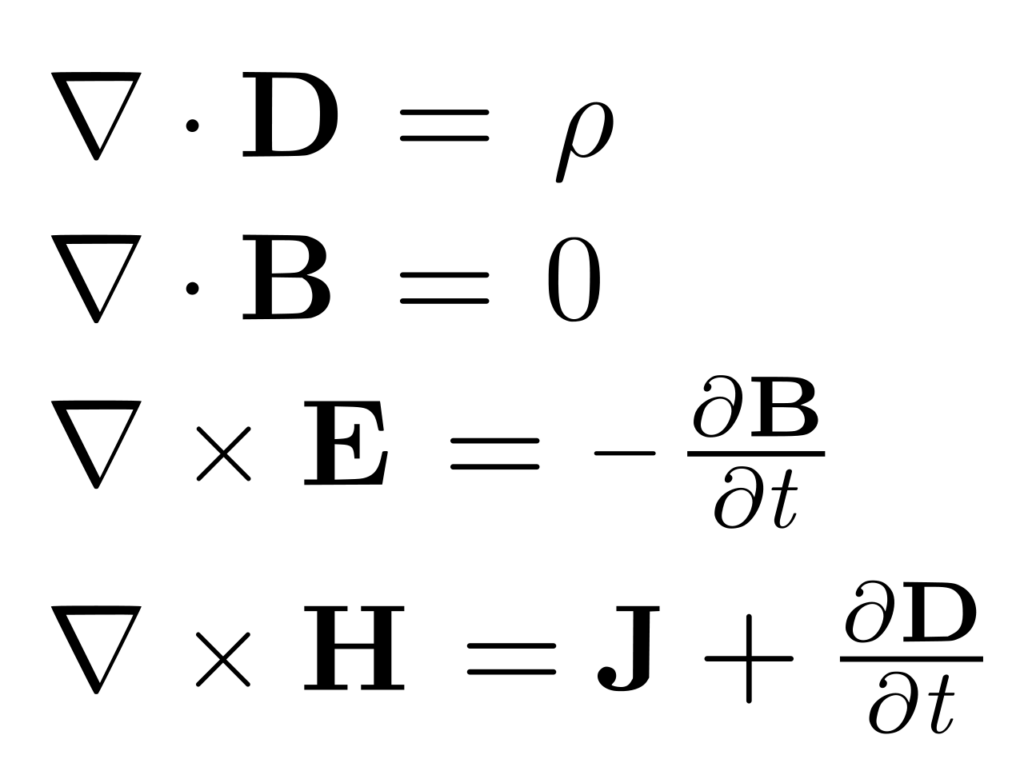
The Formulation of Maxwell’s Equations
- Gauss’s Law for Electricity (
) states that electric charges produce an electric field, and the divergence of this field is proportional to the charge density. This law is a mathematical expression of the concept that electric charge acts as a source of the electric field.
- Gauss’s Law for Magnetism (
)asserts that magnetic field lines are continuous, having neither beginning nor end. This implies there are no magnetic monopoles; instead, magnetic fields always form closed loops.
- Faraday’s Law of Induction (
) describes how a changing magnetic field over time generates an electric field. This is the principle behind electric generators and transformers.
- Ampère’s Law with Maxwell’s Addition (
) shows how an electric current or a changing electric field produces a magnetic field. Maxwell’s addition of the displacement current (
) was crucial in extending Ampère’s Law to include changing electric fields, not just steady currents.
Predicting the Existence of Radio Waves
By integrating these equations, Maxwell achieved more than a unified theory of electricity and magnetism; he predicted the existence of electromagnetic waves. Through his equations, Maxwell demonstrated that a fluctuating electric field generates a varying magnetic field and vice versa, allowing these perturbations to propagate through space as waves. He calculated the speed of these waves to be approximately 3×108 meters per second, the speed of light, suggesting that light itself is a form of electromagnetic radiation.
The Conceptual Leap
Maxwell’s leap from the understanding of static and dynamic fields to the propagation of electromagnetic waves was revolutionary. Before Maxwell, the phenomena of electricity and magnetism were largely considered in the context of forces between charges or currents, either at rest or in steady motion. Maxwell introduced the concept that changes in the electromagnetic field could propagate through space, independent of charges or currents, as waves. This insight bridged the gap between electromagnetism and optics, providing a unified description of light as an electromagnetic wave and laying the groundwork for the electromagnetic theory of light.
This conceptual leap not only validated the wave nature of light but also opened the door to the vast spectrum of electromagnetic radiation beyond visible light, including radio waves. Maxwell’s theoretical prediction of electromagnetic waves was a cornerstone for the later experimental discoveries and technological innovations that harnessed the power of the electromagnetic spectrum, fundamentally transforming communication and leading to the development of radio, radar, and microwave technology.
Experimental Validation
The theoretical predictions made by James Clerk Maxwell regarding electromagnetic waves awaited experimental confirmation for two decades until Heinrich Hertz undertook a series of groundbreaking experiments in the late 1880s. Hertz’s work not only provided the first direct evidence of radio waves but also marked a pivotal moment in the history of physics by bridging the gap between theoretical predictions and empirical evidence.
Heinrich Hertz’s Contribution
In 1886, Heinrich Hertz, a German physicist, embarked on a series of experiments aimed at detecting electromagnetic waves. Using a simple apparatus consisting of a spark gap transmitter and a loop wire receiver, Hertz was able to produce and detect electromagnetic waves in the laboratory. He observed that when an electric spark was generated in the transmitter, a corresponding spark could be detected in the receiver, even when it was several meters away. This phenomenon occurred without any visible connection between the two, indicating the presence of electromagnetic waves traveling through the air.
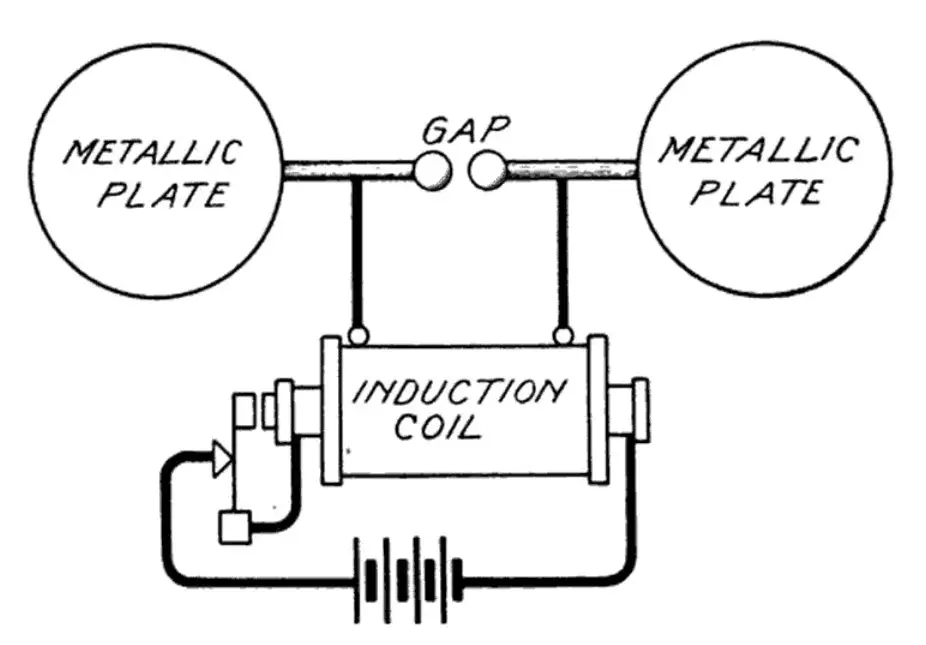
Hertz’s experiments varied the dimensions of his apparatus and demonstrated that these waves could be reflected by metallic surfaces, refracted by prisms, and diffracted around obstacles, much like light waves. By measuring the velocity and wavelength of these waves, Hertz confirmed that they traveled at the speed of light, a key prediction of Maxwell’s equations. Moreover, Hertz was able to determine the wavelength of the waves directly from his experiments, providing quantitative verification of Maxwell’s theory.
The Impact of Hertz’s Experiments
The implications of Hertz’s experiments were profound, as they provided the first tangible evidence that electromagnetic waves, as predicted by Maxwell, did indeed exist and exhibited behaviors analogous to those of light. Hertz’s work demonstrated the practical realization of Maxwell’s theoretical predictions, solidifying the electromagnetic theory of light and significantly advancing the understanding of the electromagnetic spectrum.
Hertz’s discovery of radio waves opened up new avenues for research and technological development. While Hertz himself did not immediately grasp the practical applications of his discovery, his experiments laid the foundational principles for the development of wireless communication technologies. The verification of Maxwell’s predictions through Hertz’s experiments transformed the abstract concept of electromagnetic waves into a tangible scientific reality, catalyzing further explorations into the nature of electromagnetism and paving the way for the invention of radio, radar, and other technologies that rely on the transmission and reception of electromagnetic waves.
In essence, Hertz’s experimental validation of Maxwell’s electromagnetic theory represented a landmark achievement in physics, confirming the unified nature of electromagnetic phenomena and establishing the experimental basis for the wireless communication revolution that would follow in the subsequent decades.
Interdisciplinary Echoes: Maxwell’s Equations Across Physics
The influence of James Clerk Maxwell’s equations extends far beyond the realm of electromagnetism, permeating various domains of physics and illustrating the universality of his theoretical framework. The mathematical beauty and comprehensive nature of Maxwell’s equations have found echoes in fields as diverse as fluid dynamics and quantum mechanics, showcasing the profound impact of Maxwell’s work on the broader landscape of physical science.
Similarities in Other Fields
Fluid Dynamics
In fluid dynamics, the equations governing the flow of fluids bear a remarkable resemblance to Maxwell’s equations in form and structure. For example, the Navier-Stokes equations, which describe the motion of fluid substances, mirror the complexity and dynamical nature of Maxwell’s equations. Both sets of equations involve partial differential equations that account for the evolution of a field (electromagnetic for Maxwell’s, velocity for Navier-Stokes) in space and time, highlighting conservation laws and wave phenomena. This analogy has spurred cross-disciplinary research, using insights from one field to solve problems in the other.

Quantum Mechanics
Maxwell’s influence extends into the quantum realm as well. The development of quantum electrodynamics (QED), the quantum theory that describes how light and matter interact, is deeply rooted in the principles of Maxwell’s electromagnetism. The equations of QED can be seen as a quantum counterpart to Maxwell’s classical equations, incorporating the principles of wave-particle duality and the quantization of the electromagnetic field. Furthermore, the Schrödinger equation, a fundamental equation in quantum mechanics, shares similarities with Maxwell’s equations in its wave description of particles and its use of differential equations to describe the evolution of wave functions over time.
The Universality of Maxwell’s Work
The mathematical form of Maxwell’s equations has transcended the specific phenomena of electromagnetism, influencing theoretical frameworks in numerous areas of physics. The elegance and generality of Maxwell’s formulation demonstrated that physical laws could be expressed as a set of interrelated differential equations, providing a blueprint for formulating theories in other domains of physics. This approach has become a cornerstone in the search for a unified theory of physics, guiding efforts to link the four fundamental forces of nature under a common theoretical framework.
Maxwell’s equations exemplify the power of mathematical physics to reveal the underlying unity in the diverse phenomena of the natural world. The ability of these equations to inspire and inform research across different fields of physics underscores the universality of Maxwell’s work. It highlights the interconnectedness of all physical laws and the ongoing quest to understand the complexity of the universe through the elegant language of mathematics. The interdisciplinary echoes of Maxwell’s equations affirm their status as one of the foundational pillars of modern physics, bridging the gap between classical theories and the quantum and relativistic paradigms that would follow.
Inventions and Discoveries Stemming from Maxwell’s Work
The profound implications of James Clerk Maxwell’s theoretical contributions have catalyzed a myriad of technological innovations and scientific breakthroughs. His prediction of electromagnetic waves laid the foundational principles for several key advancements in communication technology and theoretical physics.
Radio Technology
The path from Maxwell’s theoretical prediction of electromagnetic waves to the practical realization of radio technology epitomizes one of the most transformative periods in scientific history. At the cusp of the 20th century, inventors like Guglielmo Marconi harnessed the principles outlined by Maxwell to develop the first radio transmitters and receivers. Marconi’s successful transmission of wireless signals across the Atlantic in 1901 was a direct application of Maxwell’s theory, demonstrating the feasibility of long-distance communication without physical connective mediums. This monumental achievement not only validated Maxwell’s predictions but also inaugurated the era of wireless communication, fundamentally altering how information was transmitted and received worldwide.
Radar and Microwave Technology
Maxwell’s work further underpinned the development of radar technology during World War II, providing a crucial tool for military navigation and enemy detection. Radar systems, which emit radio waves and detect objects based on the reflected signals, rely on the principles of electromagnetic wave propagation initially described by Maxwell. The same underlying theory facilitated the post-war development of microwave technology, enabling a wide array of applications from cooking to satellite communication. The manipulation and control of electromagnetic waves, as predicted by Maxwell, have become integral to numerous aspects of modern technology, highlighting the enduring impact of his work on contemporary society.
Quantum Electrodynamics (QED)
On the theoretical front, Maxwell’s equations laid the groundwork for quantum electrodynamics (QED), the field of physics that describes how light and matter interact at the quantum level. QED represents a quantum-theoretical extension of Maxwell’s classical electromagnetism, incorporating the principles of quantum mechanics to account for the particle-like behavior of photons and their interactions with charged particles. The development of QED in the mid-20th century by physicists such as Richard Feynman, Julian Schwinger, and Shin-Ichiro Tomonaga was a monumental step forward in the quest to unify quantum mechanics with electromagnetic theory. This theoretical framework not only provided a deeper understanding of electromagnetic phenomena at the quantum scale but also contributed to the prediction and discovery of new particles and forces, showcasing the far-reaching influence of Maxwell’s original equations.

Conclusion
James Clerk Maxwell’s theoretical insights and the subsequent experimental validation of electromagnetic waves stand as one of the most significant achievements in the annals of physics. His formulation of the electromagnetic field theory, encapsulated in Maxwell’s equations, not only unified the then-disparate fields of electricity and magnetism but also predicted the existence of electromagnetic waves—foreseeing the means through which information, sound, and eventually data, would traverse the globe. This monumental contribution laid the groundwork for the technological innovations that have defined the modern era, from radio to radar, from telecommunications to quantum electrodynamics.
Maxwell’s legacy is not merely confined to the abstract realm of theoretical physics; it is deeply ingrained in the very fabric of our daily lives. The unseen waves that Maxwell predicted now connect the world in ways he could scarcely have imagined. Radio waves carry voices and music across vast distances, radar scans the skies for aircraft, microwaves cook food in minutes, and the principles underlying quantum electrodynamics drive the development of new technologies in computing and medicine. Each of these advancements, while diverse in application, shares a common origin in Maxwell’s groundbreaking work.
Reflecting on Maxwell’s contribution to science and technology, it’s clear that his theoretical insight into the existence of radio waves did more than expand our understanding of the electromagnetic spectrum; it provided the foundational stones upon which the interconnected world of today is built. Maxwell’s work exemplifies the profound impact that theoretical physics can have on practical technology and underscores the importance of scientific curiosity and innovation. As we continue to explore the mysteries of the universe and develop new technologies, the legacy of James Clerk Maxwell serves as a beacon, guiding us forward in our quest to understand the natural world and harness its forces to improve human life.
Further Exploration
The journey through Maxwell’s groundbreaking theory of electromagnetism and its profound impact on the world does not end here. For those captivated by the elegance of Maxwell’s equations and their monumental role in shaping modern physics, a wealth of avenues for further exploration awaits.
Technical Depth of Maxwell’s Equations: Readers with a keen interest in delving deeper into the mathematical beauty and complexities of Maxwell’s equations can explore advanced textbooks and resources on electromagnetism. Works that elucidate the transition from classical to quantum electrodynamics offer fascinating insights into how Maxwell’s framework evolves in the realm of quantum mechanics. Additionally, computational electromagnetics provides a hands-on approach to visualizing and solving Maxwell’s equations, offering a deeper understanding of electromagnetic wave propagation and interaction with materials.
Historical Context: The historical development of Maxwell’s theory is a rich tapestry woven with the efforts and insights of many scientists. Biographies of Maxwell himself, along with works detailing the lives and contributions of contemporaries such as Michael Faraday and later figures like Heinrich Hertz and Guglielmo Marconi, shed light on the human story behind these scientific advances. Understanding the challenges, debates, and eureka moments that paved the way for Maxwell’s equations enriches the appreciation of their significance.
Influenced Work and Inventors: Exploring the stories of physicists and inventors whose work was directly influenced by Maxwell’s theory offers a window into the ongoing legacy of his equations. From the development of radio technology to the cutting-edge research in quantum electrodynamics, the lineage of Maxwell’s influence spans a diverse array of fields and applications. Biographies and historical accounts of figures such as Albert Einstein, who extended the implications of electromagnetism to relativity, and quantum physicists who have taken Maxwell’s baton into the quantum realm, provide inspiration and insight into the evolution of physical theories.
Beyond Physics: Maxwell’s impact extends beyond physics, influencing areas such as electrical engineering, information technology, and even philosophy of science. Exploring how Maxwell’s equations have been applied in designing communication systems, satellites, and other technologies highlights the practical applications of theoretical physics. Additionally, discussions on the philosophy of science, focusing on the unification of natural laws and the interplay between theory and experiment, can provide a broader context for Maxwell’s work.
For those intrigued by the depth and breadth of Maxwell’s contributions, the journey of exploration is boundless. Engaging with these topics not only deepens one’s understanding of electromagnetic theory but also connects us more profoundly with the world, revealing the invisible forces that shape our daily lives and the universe at large.
Further Reading
Here are some books and websites to explore if you are interested in learning more about Maxwell’s Equations and their legacy:
- “A Treatise on Electricity and Magnetism” by James Clerk Maxwell: Maxwell’s original work, where he lays out his theory of electromagnetism. It’s a foundational text for anyone deeply interested in his equations and their derivation.
- “The Man Who Changed Everything: The Life of James Clerk Maxwell” by Basil Mahon: This biography provides insight into Maxwell’s life, his discoveries, and the impact of his work on modern physics.
- “Introduction to Electrodynamics” by David J. Griffiths: A widely used textbook in undergraduate physics courses, offering clear explanations of electromagnetic theory, including Maxwell’s Equations.
- “Quantum Electrodynamics” by Richard P. Feynman: For those interested in the quantum mechanics aspect and how Maxwell’s work influenced QED, Feynman’s lectures and books are an invaluable resource.
- “Electromagnetic Field Theory Fundamentals” by Bhag Singh Guru and Hüseyin R. Hiziroglu: This book provides a comprehensive introduction to electromagnetic field theory, emphasizing the importance of Maxwell’s Equations.
- “Faraday, Maxwell, and the Electromagnetic Field: How Two Men Revolutionized Physics” by Nancy Forbes and Basil Mahon: An accessible account of how Faraday’s experimental work and Maxwell’s theoretical framework came together to form the basis of classical electromagnetism.
- IEEE Xplore Digital Library: The IEEE Xplore Digital Library contains numerous papers, articles, and resources on the application and impact of Maxwell’s Equations in various fields of engineering and technology.
- arXiv.org: For pre-print papers and cutting-edge research related to Maxwell’s Equations and their applications in both classical and quantum physics.
- “Principles of Quantum Mechanics” by R. Shankar: Offers insights into the principles of quantum mechanics, with discussions on how Maxwell’s work influenced the development of quantum theories.
- The Feynman Lectures on Physics, Vol. 2: Specifically focused on electromagnetism and radiation, these lectures by Richard Feynman offer profound insights into the subject in an accessible manner, demonstrating the continued relevance of Maxwell’s Equations.
- Wikipedia’s James Clerk Maxwell page offers an overview of his life, scientific contributions, and the significant impact of his work on the field of physics, especially his theory of electromagnetic radiation and Maxwell’s equations.
- Magnet Academy’s biography of Maxwell highlights his theoretical work on electromagnetism and light, detailing his life from early education to his significant scientific contributions.
- Britannica’s detailed biography focuses on Maxwell’s life, providing context on his education, personal life, and detailed descriptions of his contributions to physics.
- Physics LibreTexts on Maxwell’s Equations and Electromagnetic Waves dives into the technical aspects of Maxwell’s equations, providing a mathematical and conceptual understanding of his contributions to electromagnetism.
- Lumen Learning’s exploration of Maxwell’s Predictions and Hertz’s Observations discusses Maxwell’s prediction of electromagnetic waves and the experimental confirmation by Heinrich Hertz, solidifying Maxwell’s theories.
- Engineering and Technology History Wiki’s “Maxwell’s Equations” delves into Maxwell’s return to electromagnetism, his development of a new theory, and the subsequent formulation of Maxwell’s equations.
- Molecular Expressions’ timeline and contributions of Maxwell provides a narrative of Maxwell’s life, detailing his early fascination with geometry, his educational background, and his profound contributions to electromagnetism and physics.